
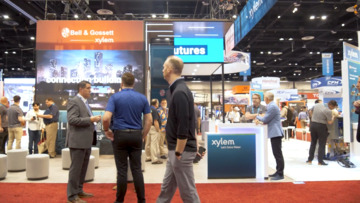
Bell & Gossett Illustrates Path to Net-zero at AHR Expo
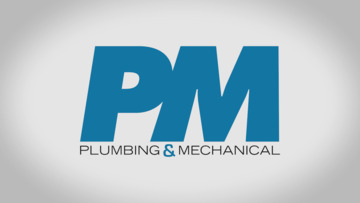
Parkway Construction Services chose REHAU RAUPEX piping with EVERLOC+ fittings for their plumbing renovation at Meharry Medical College, significantly reducing material and labor costs.
Copyright ©2025. All Rights Reserved BNP Media.
Design, CMS, Hosting & Web Development :: ePublishing