
Figure 1.
For several decades, wet-rotor circulators have captured market share in North America against traditional three-piece circulators. They’ve been increasingly used for circuit-by-circuit flow control in zoned systems, and, as such, have displaced the more traditional approach of a single system circulator and zone valves. Manufacturers have refined wet-rotor circulators to the point where they are mass-produced, reliable, virtually maintenance free, and very price competitive. Currently, about three million wet rotor circulators with input power ratings of 250 watts or less are installed in North American hydronic systems each year.
Does all this suggest that wet rotor circulators have reached a point where future improvements are not possible? Absolutely not! Improving the efficiency and versatility of wet rotor circulators will be one of the most important ongoing efforts in the hydronics industry. The primary goal is to develop products that can deliver the same superior comfort and stable operation as their predecessors, but use far less electrical energy in the process.
Another goal will be to expand the versatility of a given circulator, and reduce the need for production and stocking of many different variants and sizes. These goals will require more than a three-speed switch or internal check valve. They will require major redesign that addresses all possibilities for improvement in both hydrodynamic and electrical performance. This article will take a look at where things stand.
Prime Movers
For decades, wet rotor circulators have used permanent split capacitor (PSC) motors. Although inexpensive and reliable, PSC motors do have limitations. One of them is low starting torque because these motors do not have a start capacitor or centrifugal start switch. When used in circulators, this characteristic usually shows up as a “stuck rotor” condition, often manifesting itself after the circulator has been inactive for several months.It often results from particulates in the flow stream becoming lodged in the wet-rotor assembly and generating sufficient restraint that the motor cannot start. The traditional solution is to open the end cap, insert a screwdriver, and manually rotate the rotor to loosen it up. Simple, yes, but today each service call to free a stuck rotor ends up costing more than the circulator itself.
Another increasingly conspicuous issue with PSC-powered circulators is low wire-to-water efficiency (e.g., the ability to convert electrical energy into head energy and impart that energy to the flow stream). The relationship between wire-to-water efficiency and pump curve for a typical wet rotor zone circulator with a PSC motor is shown inFigure 1.
In this case, the circulator’s maximum wire to water efficiency is only about 22%. This implies the other 78% of electrical input wattage is converted directly to heat. This peak efficiency occurs when the circulator is operated near the middle of its pump curve. If the system forces the circulator to operate near either end of its pump curve, the resulting wire-to-water efficiency may only be in the single digit range. Would we be satisfied if the boilers we currently specify converted similar percentages of their input energy to useable heat?
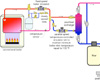
Figure 2.
Gaining Intelligence
Standard wet rotor circulators with PSC motors can operate over a wide range of speed. Doing so requires a variable frequency drive that operates according to some predetermined control algorithm. An example of such a device is an injection mixing controller that regulates the speed of a standard wet rotor circulator based on the water temperature supplied to a hydronic distribution system.One might describe a standard wet rotor circulator operated by an external speed controller as “dumb,” because the circulator itself doesn’t contain the necessary logic and circuitry to operate as a stand-alone entity. Its speed is completely determined by the signal received from the external variable frequency drive.
Wet rotor circulators with PSC motors and built-in variable speed drives are also available. They respond to an external 2-10 volt DC or 4-20 milliamp control signal. In this case, whatever varies the 2-10 volt DC or 4-20 milliamp signal holds full control over the circulator’s speed. Such circulators are sometimes used for flow regulation through terminal units and managed by a building automation system.
The next level of sophistication adds intelligence in combination with the variable speed drive. In essence, the “intelligence” that used to be housed externally to the circulator is now onboard. These specialty circulators can be used for specific tasks, such as:
- 1. Maintaining a user-specified temperature at some location in a
system by varying flow rate. An example is using an intelligent variable speed
circulator as a “thermal clutch” to protect a conventional boiler from
sustained flue gas condensation, such as for the pool heating system shown in
Figure 2.
2. Maintaining a user-defined temperature differential across some portion of the system. An example is maintaining a set temperature drop around a primary loop in a primary/secondary system as various secondary circuits turn on and off.
3. Operating as a hot water injection device to a low temperature distribution system based on either a user-specified setpoint temperature or outdoor reset control curve.
Underneath the added smarts, these devices are still wet rotor circulators with PSC motors. Although some electrical energy savings will result from reduced operating speed, their wire-to-water efficiency is still low relative to what’s possible with newer technology.

Figure 3.
The stator poles shown inFigure 3act like four energetic kids pushing and pulling a merry-go-round to keep it spinning. Each kid grabs an approaching handlebar, pulls on it for a fraction of a second, and as soon as it passes by pushes on it for another fraction of a second.
ECM-powered circulators generate about four times more starting torque compared to a PSC-based wet rotor circulator of comparable size. With this technology you can pretty much forget about stuck rotors after prolonged shut downs.
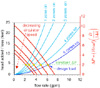
Figure 4.
Multiple Personalities
Just as a computer can run different software, the controller in a smart circulator can execute different instruction sets. This allows the circulator to operate in different modes depending on how it’s applied. In one system, the circulator can operate as a constant differential pressure device. In another system, it might operate as a temperature setpoint controller. In still another installation, it might serve as a variable speed mixing pump. The possibilities are far reaching, and allow a single circulator to potentially replace several first generation variable speed circulators that only have one or two operating modes.Two of the most useful control modes apply to systems where the variable speed circulator supplies a system in which flow through each zone is regulated by valves. One of those modes is called constant differential pressure control, and is illustrated inFigure 4. During commissioning, the circulator is set for the head (or differential pressure) required at design load conditions (when all zone valves are open).
When a zone valve closes or modulates to reduce flow, the circulator internally senses the increase in differential pressure about the setpoint value and quickly responds by reducing motor speed to cancel out this “attempted” change. This allows the operating point (e.g., the intersection between the pump curve and the current system head loss curve) to track along a horizontal line.
Constant head implies constant differential pressure. When a zone valve closes or modulates, the other zones don’t “feel” any change in differential pressure, and, thus, their flow rates remain stable. The overall effect is akin to providing “cruise control” for differential pressure. It’s what system designers have approximated in the past using differential pressure bypass valves. However, with smart variable speed circulators we no longer need bypass valves, and we get significant electrical energy savings to boot.
The constant differential pressure control mode is ideally suited for systems using valve-based zoning, and where the majority of the head loss occurs in the branch circuits and minimal head loss occurs in the common piping. An example is a manifold distribution system with short and generously sized common piping, as shown in Figure 4.
Note that the higher flow resistance boiler has been isolated on its own circuit with its own circulator. A pair of closely spaced tees is used to couple the boiler circuit to the distribution system. If a boiler with low flow resistance were used, there would be no need for the separate boiler circuit or boiler circulator. This situation is shown inFigure 5and is also well-suited to circulators with a constant differential pressure mode.
Another operating mode for a smart circulator serving a distribution system with valve-based zoning is called proportional differential pressure control.Figure 6shows the response of the circulator when set for this mode.
This operating mode is well suited to systems with supply and return mains that create significant head loss. An example is the 2-pipe reverse return system shown in Figure 6.
As with constant differential pressure control, the installer sets the circulator for the head needed at design load. When a zone valve closes or modulates, the circulator again senses an increase in differential pressure and responds by reducing motor speed. However, in the proportional differential pressure mode, the speed is reduced so the operating point tracks along a sloping line rather than the horizontal line used for constant differential pressure control.
At zero flow rate, the circulator produces one half the head it generates at design load. This mode is a better “fit” to the flow versus head loss response of this type of system when the goal is to maintain stable flow rates through the individual zones, regardless of which zones are active at a given time.

Figure 5.
Performance Plus
Predicting the seasonal electrical energy use of any circulator involves assumptions such as how many hours does the circulator run, and of those hours, where does the circulator operate on its pump curve and wire-to-water efficiency curve? This gets even more complicated with variable speed circulators that must respond to constantly changing system operating conditions.To provide a standardized means of comparison, the European manufacturers association Europump uses a predefined “duration curve” that reflects the typical flow rate conditions that exist in a zoned hydronic system over a full heating season. The duration curve gives the hours per heating season that the flow in the reference system is at or above a stated percentage of full design load flow.
For example, the reference system is at 50% or more of design flow rate a bit over 1,000 hours per season. A simplified stepwise approximation of this load profile is used to determine the required operating speed and associated energy input of a variable speed circulator over an assumed season.
Results of such simulations show that properly applied ECM-based pressure regulated circulators can reduce seasonal electrical use by 60% to 75% relative to fixed speed circulators of equivalent peak pumping ability. That’s a phenomenal achievement that often reduces the overall operating cost of even small circulators by thousands of dollars over a 20-year service life.

Figure 6.
What's Available
In North America, the current players in the ECM-based variable speed pressure regulated circulator market include Grundfos, Laing and Wilo. In the residential market, all three of these companies have models that are either currently available or very soon will be available. They range from approximately 35 to 59 watts peak input power and have minimum operating input wattages in the range of 6 watts. To facilitate easy retrofitting, expect all these models to soon be available with standard 2-bolt flanges and 6-3/8” lengths identical to current wet rotor circulators of similar capacity.Both Grundfos and Wilo currently offer “families” of ECM-based circulators available for light commercial and medium commercial size installations. Each family includes several models with different upper and lower capacities. In North America, these circulators are currently configured to operate on single phase 240 VAC power input. They are also set up for standard ANSI 2-bolt and 4-bolt flanges.
Ready for Duty
Now that variable speed ECM-based circulators are available in North America, they will steadily gain market from their fixed speed predecessors, just as mod/con boilers have gained market share from on/off boilers. Their improved wire-to-water efficiency and intelligent operating modes make them one of the “greenest” design options currently available to hydronic system designers.Building owners benefit from their use through lower operating cost. Installers and wholesales benefit from their versatility. Look for additional intelligent variable speed circulator offerings to enter the North American market in the near future. Get ready to apply them and reap the benefits.